Knitted Cell Scaffolds
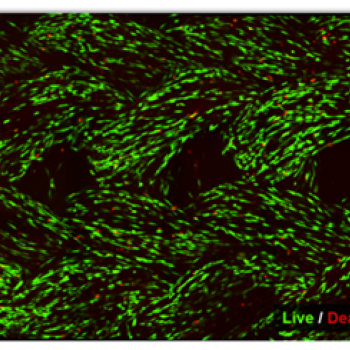
Tissue engineering seeks to restore, maintain, or enhance damaged biological tissues by utilizing scaffolds that facilitate cell growth and tissue regeneration. A fundamental requirement in scaffold design is the ability to replicate the biomechanical properties of natural tissues, ensuring an environment that supports cellular function and integration. There is an increasing demand for scaffolds that offer both elasticity and anisotropy tailored to specific tissue types, such as skin, bone, muscle, and ligaments. Achieving this level of customization necessitates advanced materials and fabrication techniques that can closely mimic the mechanical behavior and structural complexity of various anatomical regions, thereby promoting compatibility and effective integration with the body’s existing systems. However, current scaffold technologies often fall short in meeting these stringent requirements. Conventional materials like collagen, fibrin, and hydrogels typically fail to replicate the intricate stress and strain responses characteristic of natural tissues, such as the initial uncrimping and transition to fiber stretching phases. This limitation can result in inadequate mechanical support, leading to issues like cell detachment and reduced scaffold durability under physiological conditions. Additionally, existing scaffolds generally offer limited flexibility in adjusting elasticity and anisotropy, restricting their applicability across a broad range of tissue engineering applications. These shortcomings hinder effective tissue repair and regeneration, highlighting the need for more sophisticated scaffold solutions that can better emulate the complex biomechanical environment of native tissues.
Technology Description
Knitted cell scaffolds replicate natural tissue biomechanics by incorporating three distinct stress/strain phases: initial uncrimping, transition, and fiber stretching. Made from biocompatible fibers without additional supports, these scaffolds are customizable for elasticity and anisotropy through variations in knit patterns, stitch length, filament dimensions, yarn composition, and materials. Manufactured via fine-gauge knitting machines, they are sterilized with ethanol or isopropyl alcohol, ultraviolet treatment, or ethylene oxide. The scaffolds can be primed by over-stretching or cyclic stretching, and feature controllable degradation rates based on polymer type. They can be shaped to fit various anatomical needs, supporting diverse cell types for cartilage, fat, skin, muscle, bone, tendon, and ligament repair. This technology is distinguished by its ability to accurately mimic natural tissue biomechanics with a unique three-region mechanical response, surpassing conventional materials like collagen or hydrogels. Precise control over mechanical properties is achieved by adjusting multiple knitting parameters, allowing tailored elasticity for diverse tissues from skin to bone. Additionally, the scaffolds support various cell types and can be surface-modified to enhance cell attachment and growth. Customizable degradation rates and anatomical shapes further enhance their versatility for tissue engineering applications, ensuring effective tissue regeneration and integration. This combination of biomechanical fidelity and customization sets these scaffolds apart in the field.
Benefits
- Mimics natural tissue biomechanical properties with distinct stress/strain phases
- Features fully customizable elasticity and anisotropy for various tissue types
- Uses biocompatible fibers without additional support structures
- Offers controllable degradation rates tailored to specific healing timelines
- Supports a wide range of cell types, enhancing tissue regeneration
- Adapts scaffold shapes and patterns to fit diverse anatomical needs
- Facilitates improved cell attachment and growth through surface modifications
Potential Use Cases
- Cartilage repair
- Muscle, bone, and tendon tissue regeneration
- Skin reconstruction