Radar and communications system extends signal range at millimeter-wave frequencies
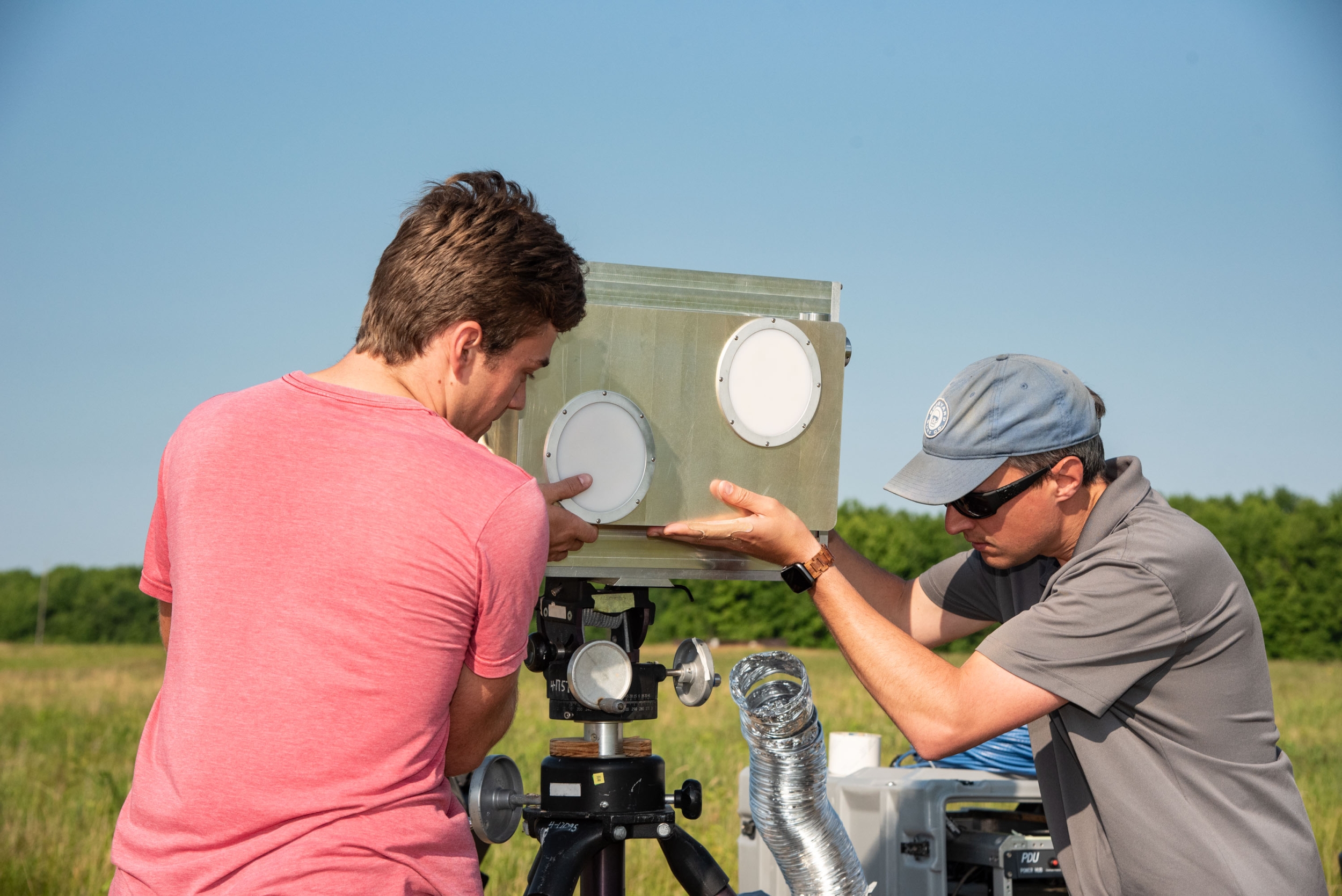
A team from MIT Lincoln Laboratory has built and demonstrated the Wideband Selective Propagation Radar (WiSPR), a system capable of seeing out various distances at millimeter-wave (MMW) frequencies. Typically, these high frequencies, which range from 30 to 300 gigahertz (GHz), are employed for only short-range operations. Using transmit-and-receive electronically scanned arrays of many antenna elements each, WiSPR produces narrow beams capable of quickly scanning around an area to detect objects of interest. The narrow beams can also be manipulated into broader beams for communications.
"Building a system with sufficient sensitivity to operate over long distances at these frequencies for radar and communications functions is challenging," says Greg Lyons, a senior staff member in the Airborne Radar Systems and Techniques Group, part of Lincoln Laboratory's ISR Systems and Technology R&D area. "We have many radar experts in our group, and we all debated whether such a system was even feasible. Much innovation is happening in the commercial sector, and we leveraged those advances to develop this multifunctional system."
The high signal bandwidth available at MMW makes these frequencies appealing. Available licensed frequencies are quickly becoming overloaded, and harnessing MMW frequencies frees up considerable bandwidth and reduces interference between systems. A high signal bandwidth is useful in a communications system to transmit more information and in a radar system to improve range resolution (i.e., ability of radar to distinguish between objects in the same angular direction but at different distances from the radar).
The phases for success
In 2019, the Laboratory team set out to assess the feasibility of their MMW radar concept. Using commercial off-the-shelf radio-frequency integrated circuits (RFICs), which are chips that send and receive radio waves, they built a fixed-beam system (only capable of staring in one direction, not scanning) with horn antennas. During a demonstration on a foggy day at Joint Base Cape Cod, the proof-of-concept system successfully detected calibration objects at unprecedented ranges.
"How do you build a prototype for what will eventually be a very complicated system?" asks program manager Christopher Serino, an assistant leader of the Airborne Radar Systems and Techniques Group. "From this feasibility testing, we showed that such a system could actually work and identified the technology challenges. We knew those challenges would require innovative solutions, so that's where we focused our initial efforts."
WiSPR is based on multiple-element antenna arrays. Whether serving a radar or communications function, the arrays are phased, which means the phase between each antenna element is adjusted. This adjustment ensures all phases add together to steer the narrow beams in the desired direction. With this configuration of multiple elements phased up, the antenna becomes more directive in sending and receiving energy toward one location. (Such phased arrays are becoming ubiquitous in technologies like 5G smartphones, base stations, and satellites.)
To enable the tiny beams to continuously scan for objects, the team custom-built RFICs using state-of-the-art semiconductor technology and added digital capabilities to the chips. By controlling the behavior of these chips with custom firmware and software, the system can search for an object and, after the object is found, keep it in "track" while the search for additional objects continues — all without physically moving antennas or relying on an operator to tell the system what to do next.
"Phasing up elements in an array to get gain in a particular direction is standard practice," explains deputy program manager David Conway, a senior staff member in the Integrated RF and Photonics Group. "What isn't standard is having this many elements with the RF at millimeter wavelengths still working together, still summing up their energy in transmit and receive, and capable of quickly scanning over very wide angles."
For the communications function, the team devised a novel beam alignment procedure.
"To be able to combine many antenna elements to have a radar reach out beyond typical MMW operating ranges — that's new," Serino says. "To be able to electronically scan the beams around as a radar with effectively zero latency between beams at these frequencies — that's new. Broadening some of those beams so you're not constantly reacquiring and repointing during communications — that's also new."
Another innovation key to WiSPR's development is a cooling arrangement that removes the large amount of heat dissipated in a small area behind the transmit elements, each having their own power amplifier.
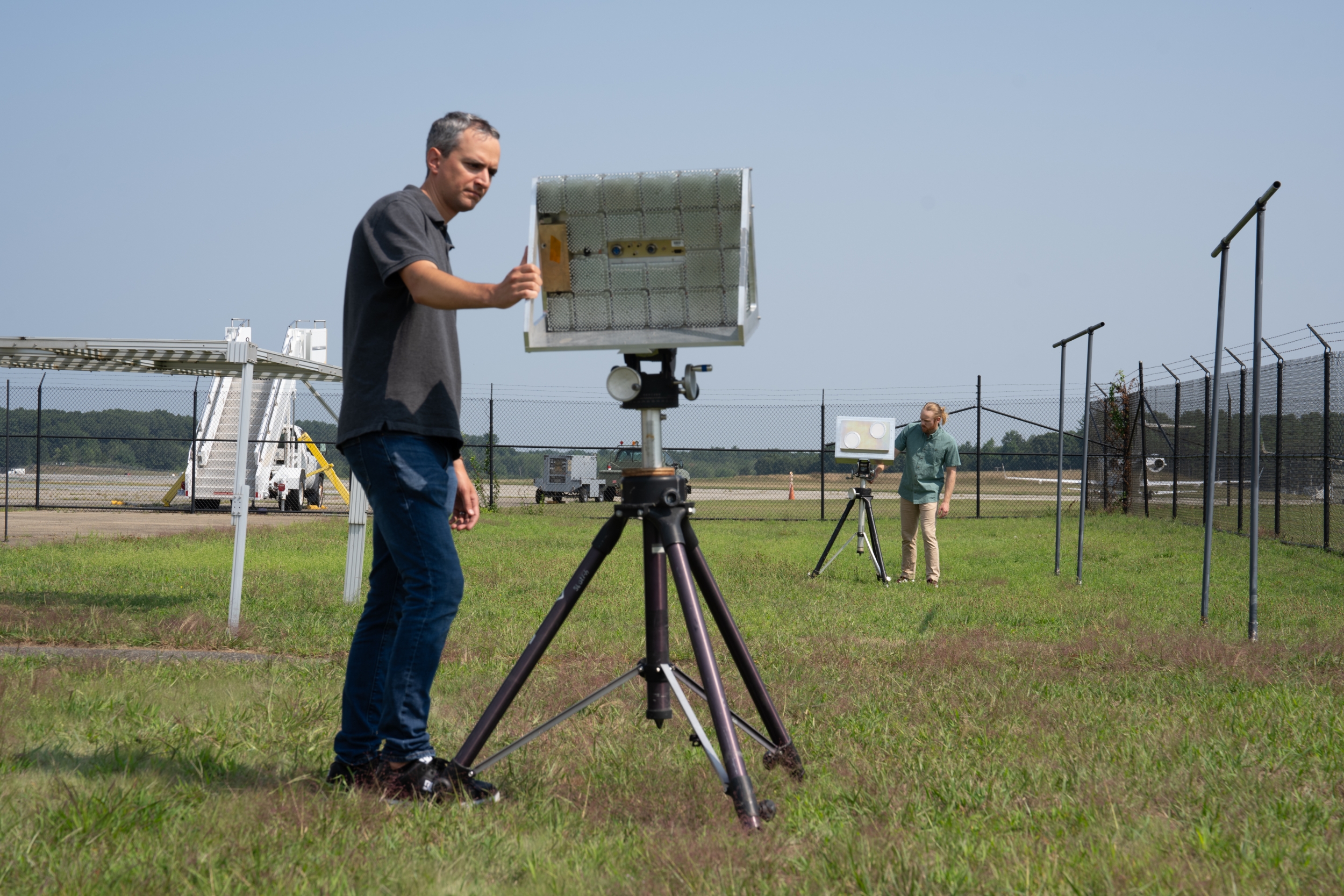
Last year, the team demonstrated their prototype WiSPR system at the U.S. Army Aberdeen Proving Ground in Maryland, in collaboration with the U.S. Army Rapid Capabilities and Critical Technologies Office and the U.S. Army Test and Evaluation Command. WiSPR technology has since been transitioned to a vendor for production. By adopting WiSPR, Army units will be able to conduct their missions more effectively.
"We're anticipating that this system will be used in the not-too-distant future," Lyons says. "Our work has pushed the state of the art in MMW radars and communication systems for both military and commercial applications."
"This is exactly the kind of work Lincoln Laboratory is proud of: keeping an eye on the commercial sector and leveraging billions-of-dollars investments to build new technology, rather than starting from scratch," says Lincoln Laboratory Assistant Director Marc Viera.
This effort supported the U.S. Army Rapid Capabilities and Critical Technologies Office. The team consists of additional members from the Laboratory's Airborne Radar Systems and Techniques, Integrated RF and Photonics, Mechanical Engineering, Advanced Capabilities and Systems, Homeland Protection Systems, and Transportation Safety and Resilience Groups.
Inquiries: contact Ariana Tantillo.
Related Links