Christine Wang is elected to the National Academy of Engineering
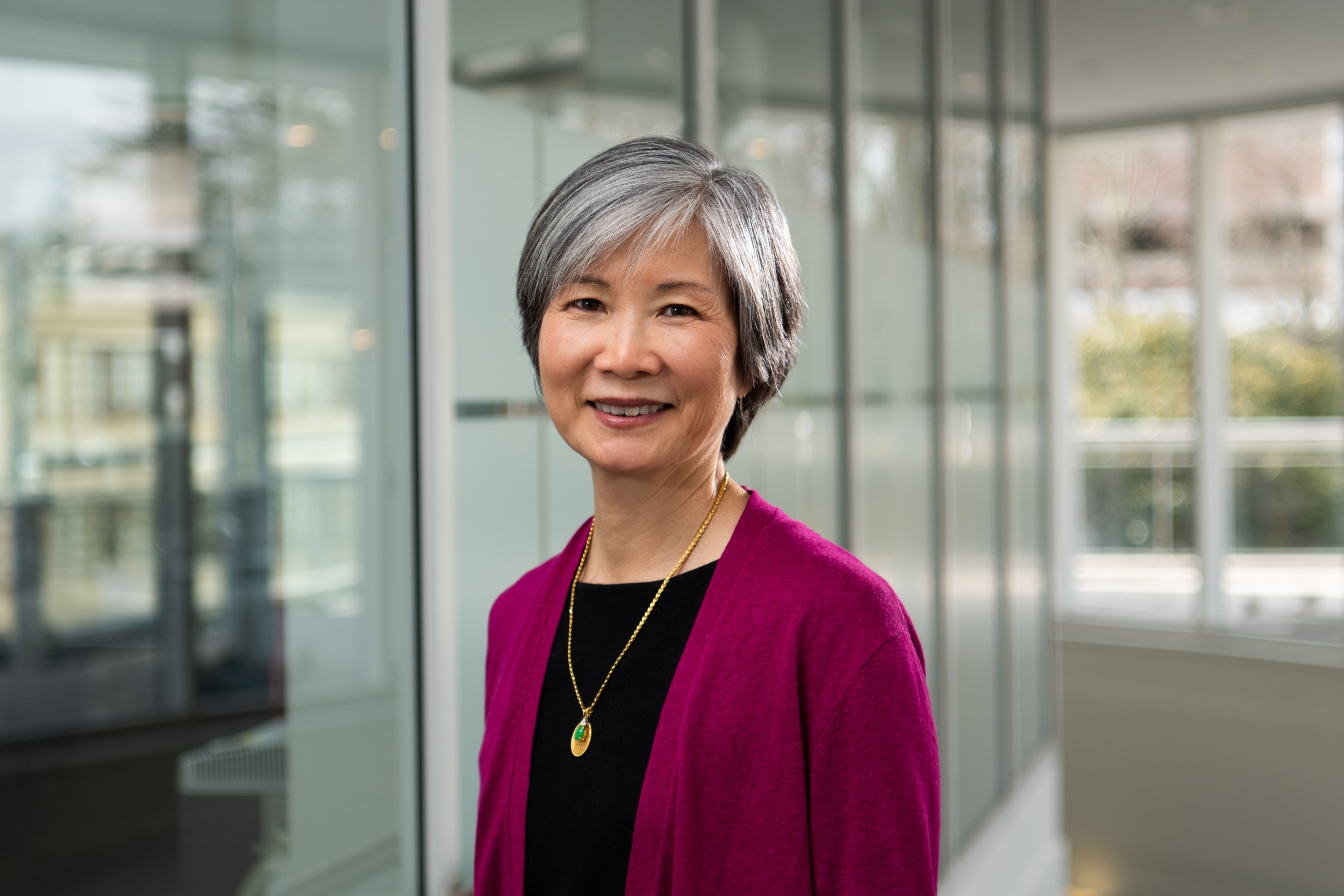
When Dr. Christine Wang came to Lincoln Laboratory in 1984, President Reagan had recently announced the Strategic Defense Initiative, also known as the "Star Wars" program, which aimed to create a missile defense system and called for developing lasers to intercept incoming nuclear missiles. The program, which would eventually be called off, faced many obstacles, but one problem was how to produce the epitaxial semiconductor materials needed for these lasers. The epitaxial materials would have to be produced controllably, reproducibly, and uniformly over large areas and with high yield.
Spurred by the initiative, Wang, with MIT and Laboratory colleagues, solved these problems by using an innovative approach to design a new kind of organometallic vapor-phase epitaxy (OMVPE) reactor. Today, Wang's design concepts are incorporated in the major large-scale commercial OMVPE reactor platforms used worldwide for the production of III-V compound semiconductor epitaxial materials needed for lasers, light-emitting diodes (LEDs), high-efficiency solar cells, high-speed electronic devices, and other electronic and optoelectronic devices.
For this work, and for her contributions to epitaxial crystal growth of III-V compound semiconductors, Wang has been elected as a member of the National Academy of Engineering (NAE). Election to the NAE is one of the highest professional distinctions conferred to an engineer.
"It is such a tremendous honor. It was a complete surprise, and it's overwhelming to be recognized for my years of work at the Laboratory," said Wang, who is now a senior staff member in the Laser Technology and Applications Group.
III-V compound semiconductors refer to elements from the "group III" and "group V" columns on the periodic table. When combined, these elements form materials with semiconducting properties. These III-V materials are unique from group IV semiconductors such as silicon because of their ability to emit light. Thus, they are used in lasers, LEDs, photodetectors, high-efficiency solar cells, and many other devices.
"Today, these semiconductors are at the heart of so many technologies that are used ubiquitously around us — they are in your smart phones, fiber communications, wireless communications, lighting with LEDs, all sorts of sensors, and so on. But at the time, in the 1980s, we didn't know the full impact of how semiconductors would change how we live today. We didn't know how to produce, or as we say 'grow', them uniformly and reproducibly and with high yield," Wang said. "You can't build any industry if your process is not controllable and reproducible."
One method to produce these III-V epitaxial semiconductors is by the OMVPE technique. Chemicals containing the group III and group V elements are fed into a hot reactor via a gas stream. These chemicals react, and atoms are deposited onto a single crystal wafer substrate, forming III-V epitaxial crystal layers. Ideally, these layers grow highly structured and uniformly on the substrate and can then be processed for use in a device.
In practice, however, epitaxial layers grown in existing reactors were far from uniform. Thus, in the mid 1980s, Wang joined the burgeoning international research efforts in OMVPE reactor design. She teamed up with Robert Brown, then a professor in chemical engineering at MIT, to model the reactor by using physical and numerical simulations combined with growth experiments.
"We went with a vertical geometry, which can inherently yield layer uniformity, and to make it more straightforward to numerically simulate, we used a straight cylindrical tube," Wang said. A key element in designing the reactor was developing a method to introduce the gases into the tube so that the gases evenly distributed throughout the tube. In addition, they identified the important roles of wafer rotation and reactor pressure in producing uniform epitaxial layers with controllability and reproducibility.
With the help of James Caunt in the Laboratory's Engineering Division, the first prototype of this newly designed OMVPE reactor was built in 1986. "The uniformity and reproducibility of the materials and the performance of gallium-arsenide quantum-well diode lasers that we demonstrated were unprecedented. It got a lot of press," Wang said. With an MIT mechanical engineering graduate student, she then developed a general framework for scaling up the capacity of this reactor in way that did not require intensive numerical simulations. This large-scale reactor was patented and the technology was transferred to several organizations. It has since become a standard tool in the multibillion-dollar compound-semiconductor industry for producing a wide array of advanced electronic and optoelectronic devices.
Beyond her work on OMVPE reactors, Wang has led the use of nonconventional chemical compounds to enable epitaxial growth of antimonide-based III-V semiconductors. She's advanced the state of the art in the epitaxial growth of gallium arsenide–, gallium antimonide–, and indium phosphide–based optoelectronic devices, including diode lasers, quantum cascade lasers, and thermophotovoltaic cells.
For the last 10 years, Wang has also been working on quantum cascade lasers emitting in the long-wave infrared wavelength region. These lasers have the most difficult device structure she has had to grow, consisting of nearly a thousand layers, some of which are less than one nanometer thick. "That's equivalent to a few atomic layers of the III-V semiconductor," Wang said. These lasers were developed in collaboration with Federico Capasso's group at Harvard University.
Wang said that collaboration has been essential to achieving results that stand out above the norm. "Epitaxial crystal growth of III-V semiconductors is a very interdisciplinary effort, and working with other materials scientists, chemical engineers, mechanical engineers, chemists, physicists, and electrical engineers with their expertise has not only been key to our work, but also has been important in building relationships with people both inside and outside the Laboratory," she said. "In particular, the ultimate metric of our epitaxial materials is device performance, and the opportunities to work side-by-side with device physicists and engineers at the Laboratory to optimize performance has been integral to my success."
Publishing her work has been important, too. Wang has authored or coauthored more than 170 publications, has been granted eight patents, and has edited one book. "Publishing, putting yourself out there, and going to conferences are some of the best ways to build relationships and show others what you can do," she added. This advice is some that she shares with the many staff and students she has mentored throughout her career.
Wang serves as the program co-chair for the 2019 International Conference on Crystal Growth and Epitaxy. She is a member of the Executive Committee for American Association of Crystal Growth, the International Advisory Committee for International Conferences on Metalorganic Vapor Phase Epitaxy, and the Electronic Materials Conference Committee. Wang is also a Fellow of the National Academy of Inventors. She earned bachelor's, master's, and doctoral degrees in materials science and engineering at MIT.
Wang joins William Delaney, Peter Moulton, Grant Stokes, and Eric Evans as current Lincoln Laboratory employees who have been elected to the NAE.