ILLUMA-T launches to the International Space Station
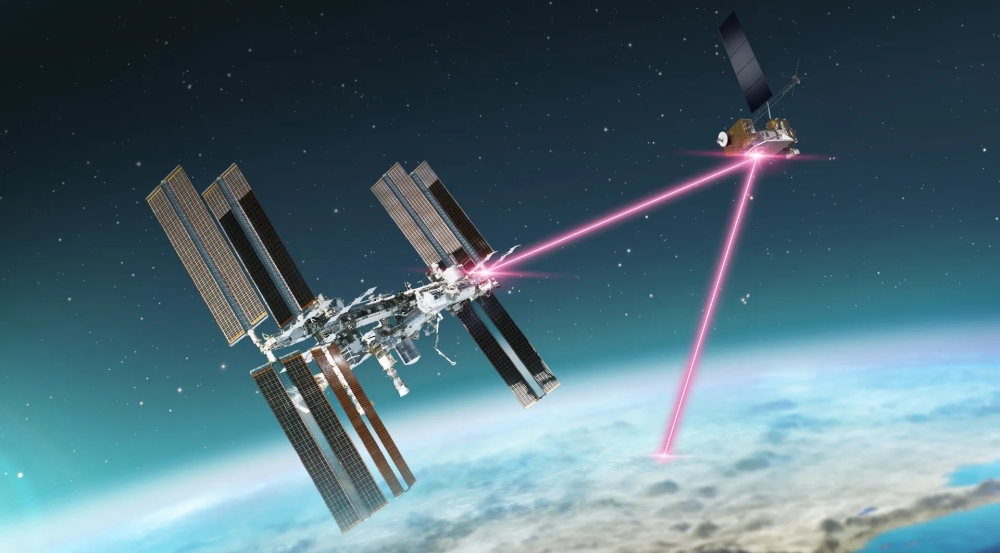
On November 9, a Lincoln Laboratory–developed laser communications terminal integrated on a NASA-built payload was launched aboard a SpaceX Falcon 9 vehicle. Cameras inside the launch vehicle enabled the Laboratory and NASA Goddard Space Flight Center team to watch as the payload headed for the International Space Station (ISS), a football-field-sized research platform orbiting Earth about 250 miles above its surface, an altitude known as low Earth orbit (LEO). On the ISS, the terminal — called ILLUMA-T (for Integrated Laser Communications Relay Demonstration [LCRD] LEO User Modem and Amplifier Terminal) — will participate in a technology demonstration to showcase the advantages of laser communications for missions in LEO. The team seeks to demonstrate that ILLUMA-T can enable high data-transmission rates from the ISS to NASA’s LCRD satellite in geosynchronous orbit (GEO) and ultimately to ground stations on Earth, and also from the ground back up to the ISS.
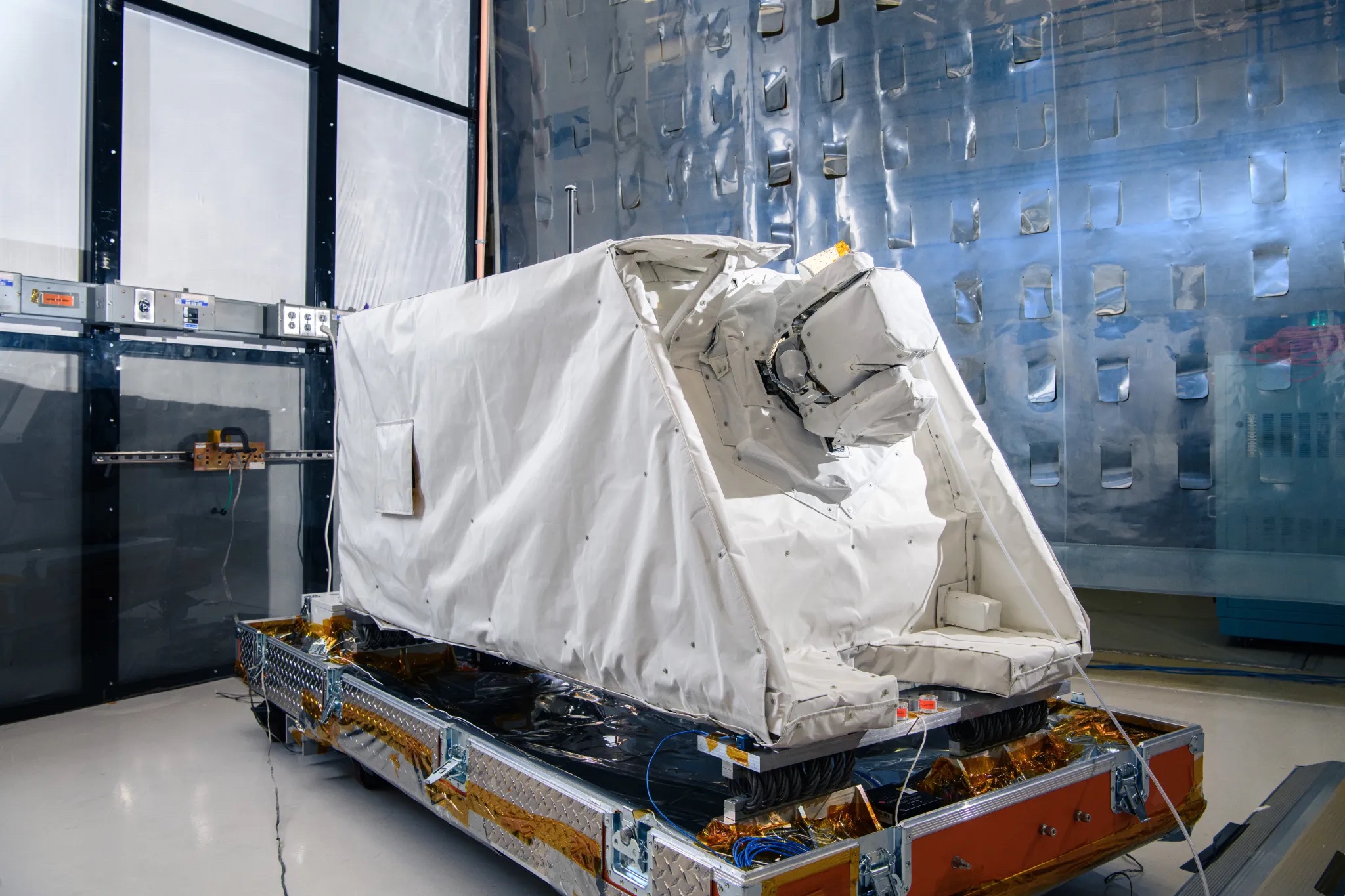
“We’re excited for ILLUMA-T and LCRD to demonstrate NASA’s first LEO-GEO optical communications relay,” says Bryan Robinson, an associate leader of the Laboratory’s Optical and Quantum Communication Technology Group. “Our close collaboration with Goddard on engineering development and operations was critical to this mission.”
Lighting the way for future space communication
Most space-based missions today use radio frequencies (RF) for communication. But infrared laser light (owing to its shorter wavelength) can transmit data at rates 10 to 100 times faster. This speedup means missions can send more data — for example, images, videos, sensor outputs, and command-and-control information — to and from space in much less time. Laser communications systems also require less volume, weight, and power than their RF counterparts, translating to lower mission costs.
NASA first performed two-way space communication with laser light instead of RF in 2013 with the Lunar Laser Communications Demonstration (LLCD), for which the Laboratory designed and built the space and ground terminals. That year, LLCD made history by transmitting data at record-breaking download and upload rates over the 239,000 miles between the Moon and Earth (more specifically, to a Laboratory-built ground station in New Mexico). As LLCD was winding down, NASA embarked on another laser communications development effort: LCRD. Goddard built the two laser communications terminals for LCRD based on the LLCD design. Since launching into GEO 22,000 miles above Earth’s surface in 2021, LCRD has been relaying data between ground stations in Hawaii (built by the Laboratory) and in California (built by Caltech’s Jet Propulsion Laboratory), with NASA conducting experiments and assessing system performance.
“The next question NASA asked as they finished LLCD and invested in LCRD was whether the LLCD terminal design could be put onto a LEO spacecraft,” Robinson says. “The ISS was always the target for that demonstration.”
Redesigning the terminal
The design of the LLCD terminal was constrained in some ways that made its transfer onto a LEO satellite difficult. The biggest constraint was related to its field of regard, or the directions in which the terminal can point when LCRD is within sight of the LEO satellite. As a LEO satellite orbits the Earth (about once every 90 minutes), the laser beam must be moved quickly to stay pointing toward a GEO satellite over all angles. However, the original terminal design was limited to about 20-degrees motion in both the vertical and horizontal directions.
“That range of motion works great in GEO because the satellite is in a fixed position; it’s not moving relative to the Earth, and the extent of Earth is within those 20 degrees,” Robinson explains. “So, you can point anywhere on Earth and you don’t have to move very fast.”
The Laboratory team started reworking the terminal design after the LLCD program completed operations in 2014. They added a two-axis gimbal (pivoted support that allows an object to rotate about an axis) capable of pointing anywhere in the hemisphere of directions as the satellite moves through LEO. The gimbal can quickly pivot to track a GEO satellite.
In addition to making the terminal design more functional, the team made it more manufacturable. While coming up with and testing initial concepts, they sought input from industry partners and tailored several aspects of the design accordingly. For example, they incorporated all the fine-pointing mechanisms — such as light-focusing lenses, tracking sensors, and fast-steering mirrors — in a backend optical assembly. In this new design, the mechanisms are slightly larger and easier to align. Such alignment is necessary to precisely point the laser beam in the desired direction for communication, and the team incorporated additional pointing-correction mechanisms to relax the alignment tolerance requirements. The assembly is separated from the telescope, which is exposed to more-extreme space environments, enabling better control of the temperature and pointing stability of the optics.
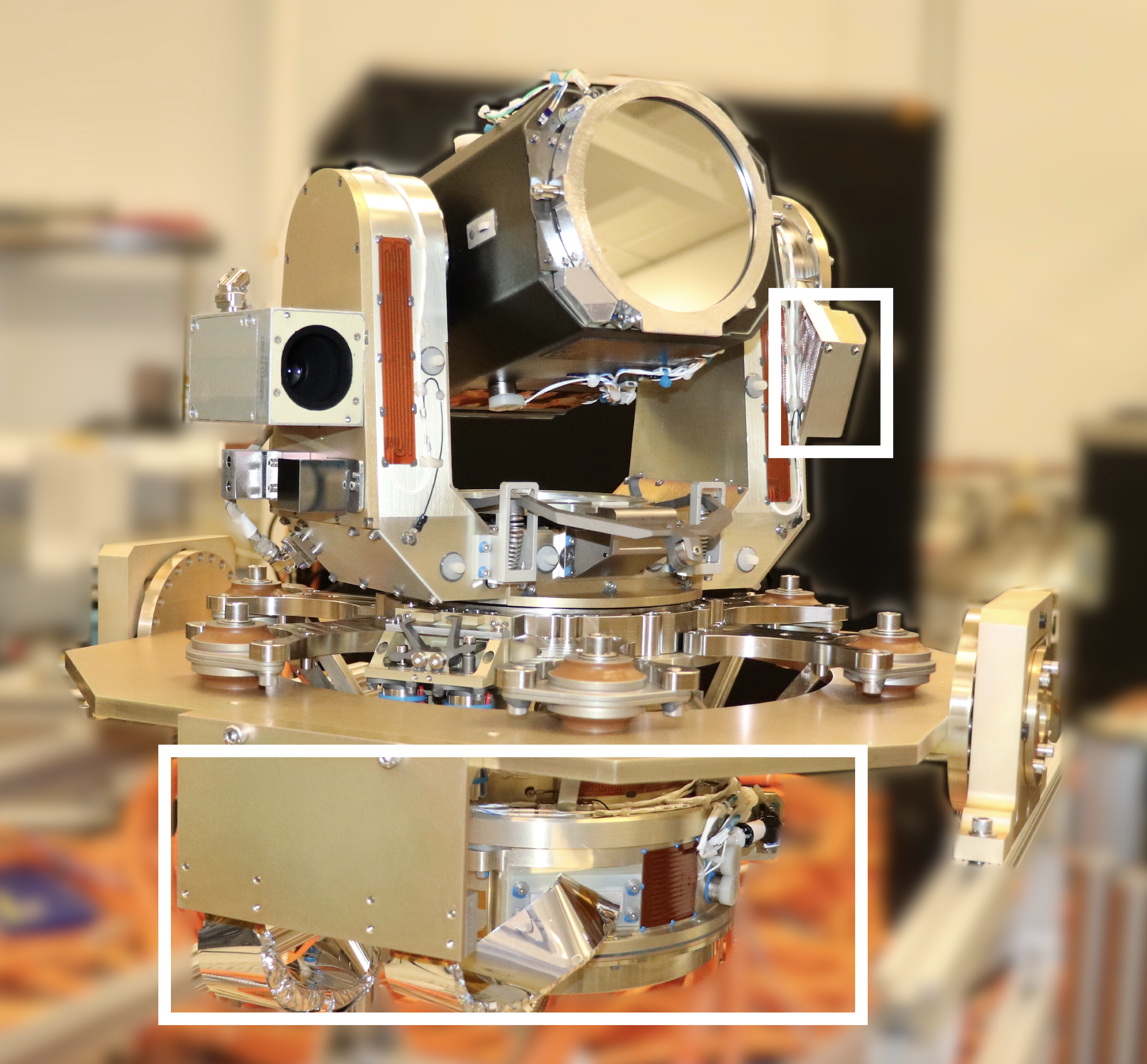
NASA then initiated an effort to put this terminal, called MAScOT (for Modular, Agile, Scalable Optical Terminal), on the ISS. ILLUMA-T is now bringing MAScOT into space for the very first time.
Embarking on a six-month mission
Over the next two weeks, ILLUMA-T, packaged in an enclosure compatible with ISS interfaces, will be installed on an external module of the ISS, the Japanese Experiment Module – Exposed Facility. Two separate cranes will lift the ILLUMA-T payload into its designated space. Thanks to cameras stationed on the ISS, the team will be able to follow the installation process, unlike previous laser communications missions like LLCD, in which the payload was launched and never seen again.
Once installation is complete, the team will power on ILLUMA-T and perform in-orbit checkouts. Following this one-month commissioning phase, they will attempt to attain first light — that is, when ILLUMA-T transmits its first beam of laser light through its optical telescope to LCRD.
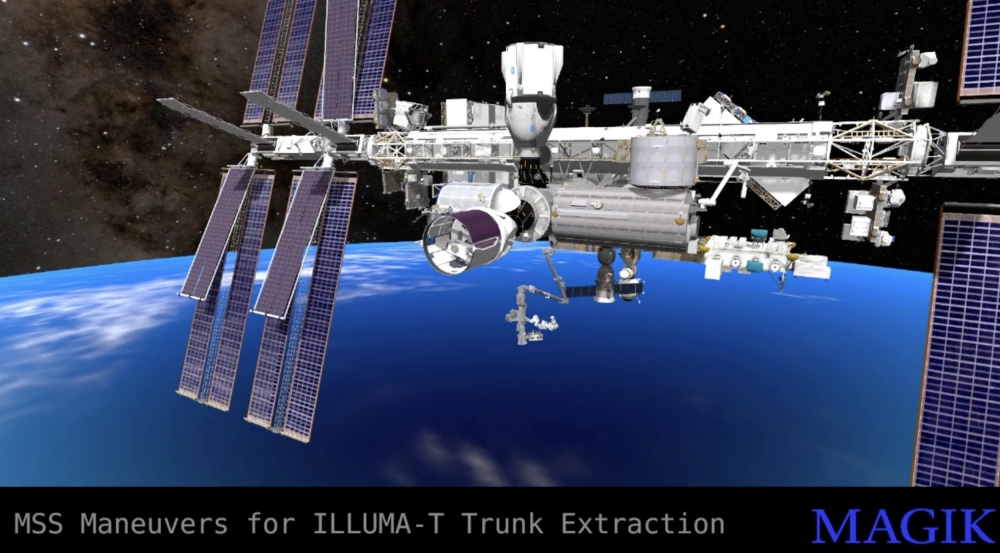
After achieving this critical milestone, the team will begin laser communications experiments to send data from ILLUMA-T on the ISS to LCRD to the ground (return direction) and vice versa (forward direction). They plan to demonstrate a return rate of 1.2 gigabits per second (Gbps) and a forward rate of 51 megabits per second (Mbps), with additional modes operating up to 155 Mbps. These return and forward rates are considerably higher (~2X and ~6X higher, respectively) than those currently provided by radio systems on the ISS and are achieved with a terminal much smaller than the RF terminal.
“High data rates are useful when astronauts are involved in the mission,” says Farzana Khatri, senior staff in the Laboratory’s Optical and Quantum Communication Technology Group. “A reliable data link from the ground to space is important for streaming internet and staying connected with the astronauts to perform telehealth, for example. Also, on the ISS are many computers, all of which need to be regularly updated and patched.”
ILLUMA-T, composed of the MAScOT optical module and a modem, is connected via Ethernet to the ISS local area network, to which computers and other experiments plug into. So, ILLUMA-T can send various kinds of data from the ISS, such as scientific measurements and system health and status indicators.
“Our goal during the experiments is to ensure the optical links work as expected and provide the defined data rates,” Robinson says.
Employing the terminal design in future missions
The experiments will span five months, after which ILLUMA-T is anticipated to deorbit from the ISS, with another system taking its place. But ILLUMA-T only represents the initial use of MAScOT. The same terminal design will be employed in the Orion Artemis II Optical Communications System (O2O). Scheduled to launch aboard NASA’s Orion spacecraft in November 2024, O2O will bring laser communications to the Moon for the human-crewed Artemis II mission. The four Artemis II astronauts will be the first humans since 1972 to take the trip to the Moon. The Laboratory team has already built and delivered the optical terminal for O2O to Kennedy Space Center, where it has been installed on Orion and is undergoing testing.
The team is now exploring how MAScOT could be integrated with a high-rate optical modem to support the Event Horizon Explorer (EHE), which requires data link rates of more than 200 Gbps. The Event Horizon Telescope (EHT) captured the first-ever image of a black hole in 2019; the EHE seeks to extend the ground-based EHT with a space-based node in GEO to capture even sharper images revealing intricate details such as halos of light formed by particles orbiting a black hole. One possibility for the high-rate optical modem is that used for the Laboratory-developed TeraByte Infrared Delivery (TBIRD) laser communications payload launched into LEO in 2022. While ILLUMA-T uses a 1.2 Gbps industry-produced modem specifically designed to operate in space and work with the signaling used by the LCRD spacecraft, TBIRD uses an augmented 100 Gbps commercial modem designed for terrestrial fiber-optic networks — the ones that power internet, telephone, and television services across the globe today. TBIRD demonstrated a successful link from LEO to the ground with a very small telescope. However, for EHE and other GEO missions, closing the much-longer link (~100X) from GEO to the ground with a high-rate commercial modem will require a larger telescope, like that of MAScOT, and higher transmit powers.
“We developed MAScOT to be more flexible than the terminals for LLCD and LCRD,” says Robinson. “Many missions now and in the future are expected to benefit from this new design.”
The ILLUMA-T payload is managed by NASA Goddard. The ISS program office at NASA’s Johnson Space Center is a partner. ILLUMA-T is funded by the Space Communications and Navigation (SCaN) program at NASA Headquarters in Washington.
Inquiries: contact Ariana Tantillo.
Related Links